Are Brain Organoids The Next Big Thing?
Abstract
Using human-derived stem cells, researchers are now able to create stable and functional 3D constructs that mimic some aspects of brain development.
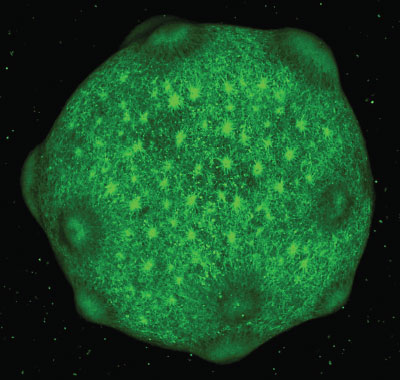
Among the various organs, the human brain is both the most complex and most inaccessible. This combination has made psychiatric research daunting, as both ethical and logistical obstacles prevent detailed analysis of living brain tissue. Postmortem samples, animal models, and brain scans offer clues about how the brain works, but each approach to studying the brain has notable limitations.
In the past decade, a new model system has been gaining steam in neuroscience—the brain organoid. These 3D constructs are grown from human stem cells and mimic many aspects of brain development. Brain organoids average the size of a tiny pea, but within that clump of cells, neurons are growing, making connections, and sending electrical signals to each other.
News stories have both hailed brain organoids’ therapeutic promise and warned of the ethical dilemma of these lab-made constructs. But experts caution there are limits to what even the most complex brain organoids can do.
For one, organoids lack blood vessels and critical supporting tissues, which limits their ability to grow past a few millimeters in length and be viable for more than a few years, even in optimal conditions.
“The general consensus is that organoids are not models of a brain but rather models for certain aspects of brain development,” Paola Arlotta, Ph.D., chair of Harvard University’s Department of Stem Cell and Regenerative Biology, told Psychiatric News. “Still, if you want to understand human development, you need human models; there are no shortcuts.”
From Organoids to Assembloids
The excitement over organoids as tools to understand mental illness can be traced back to a pair of advances by Japanese researchers. The first was the Nobel Prize–winning discovery of induced pluripotent stem cells (iPSCs) from Kyoto University’s Shinya Yamanaka, M.D., Ph.D., in 2006. Yamanaka and his group pioneered a process to reprogram adult human cells back into their stem-cell state. With this advance, researchers could now scrape a few skin cells from a living human, reprogram the cells, and watch them grow up as heart cells, lung cells, or even brain cells.
“For researchers like me interested in neurodevelopmental disorders like autism, iPSCs were a revolution,” said Flora Vaccarino, M.D., the Harris Professor of Child Psychiatry at Yale University. “In adult cells, every developmental misfire that contributed to a disorder like autism has already happened.” With iPSCs, researchers had a way to reset that developmental clock, she said.
For example, Vaccarino could grow iPSC neurons from 10 different people with autism in a Petri dish and look for similarities and differences in their growth. She could also grow 10 samples from the same person and expose each sample to different chemicals to test their effects.
Early iPSC assemblies were mainly manmade constructs grown as 2D sheets on a plate or spread over organic scaffolds to create primitive 3D tissues. However, a couple years after iPSCs burst forth, Yoshiki Sasai, M.D., Ph.D., and his lab at the RIKEN Institute in Kobe, Japan, demonstrated that under the right conditions, embryonic stem cells grown in liquid could self-organize into functional 3D constructs with multiple types of cells. With this advancement, investigators could let stem cells mature and assemble naturally. Vaccarino’s lab became the first to apply Sasai’s technique and generate 3D brain organoids from iPSCs in 2012, and three years later developed the first organoids derived from individuals with autism.
“I never thought I would become a tool developer, but when iPSCs were reported toward the end of my medical studies, I realized their potential to help us understand brain diseases,” said Sergiu Pasca, M.D., an associate professor of psychiatry and behavioral sciences at Stanford University. Pasca, who is interested in understanding autism and neurodevelopmental disorders, came to Stanford to conduct postdoctoral studies with these revolutionary cells.
Around 10 years ago, Pasca, who is also the Bonnie Uytengsu and Family Director of the Stanford Brain Organogenesis Program, shifted from the 2D to 3D. But while other groups were trying to refine the growing conditions that let stem cells naturally differentiate into multilayered brain tissue, Pasca’s team used chemical instructions to direct iPSCs to form 3D structures of a specific brain region such as cerebral cortex or striatum.
Pasca published his first study on directed organoid formation in 2015, and in the years since, this research has expanded exponentially.
“It seems like there is a new paper on brain organoids coming out every day,” said Vaccarino, who has developed nearly 600 iPSC lines from over 100 people, including people with developmental disorders and their unaffected family members.
As the process for developing brain organoids has become more routine, researchers have started to combine individual organoids into larger units known as assembloids. Pasca’s team developed the first brain assembloids in 2017, by putting together organoids of two different regions of the forebrain to form synaptic connections and communicate with each other. More recently, his lab generated assembloids with more complex connectivity, including an assembloid combining organoids of the cerebral cortex, spinal cord, and skeletal muscle. The three-part system was functional, as stimulation of the cortex organoid triggered contractions in the muscle.
Limits of Developing in a Dish
Given all the progress and excitement, it is easy to forget that organoid science is still a young and immature discipline. The initial studies focused on optimizing growth conditions and comparing organoids to postmortem brain samples to see how well they matched adult brains. Researchers have only begun detailed analyses of organoids cultured from people with psychiatric disorders in the past few years. Though experts are hopeful these models will answer some important questions, they acknowledge there are limitations to the model.
The first limitation is time. Though human organoids are reprogrammed cells grown in an artificial medium, they still follow normal developmental timeframes. “We can alter these cells a lot, but their internal clocks remain human,” said Arlotta. Thus, an organoid growing over a period of nine months will roughly mimic the steps of fetal brain development.
That these organoids work on a human timespan makes them more scientifically valid, but also means they are a slow, tedious, and expensive model system. Another current limitation of the model is that brain organoids have very limited lifespans. Some research teams have maintained organoids for up to five years, but Arnold Kriegstein, M.D., Ph.D., a professor of neurology at the University of California, San Francisco, and director of the school’s Stem Cell Center, noted that organoids may not be entirely reliable. “These systems are growing under stress in artificial environments,” he explained. “The elevated stress can change gene expression in organoid cells and degrade their cellular identity.”
Improved growing techniques could increase the stable lifespan of organoids, but no laboratory conditions reflect life within a placenta. As Vaccarino explained, when fetal brains mature, they respond to chemical signals both internal and external; where a brain cell is positioned relative to future heart or skin cells dictates its future trajectory. “I think with time we can get better at inducing some of this early brain patterning, but we could not fully recreate it,” she said.
Brain organoids also lack sensory inputs, which deprives them of the key means by which growing brains learn.
Last year, Kriegstein’s lab conducted some studies comparing gene expression of frontal cortex organoids grown in a variety of ways relative to corresponding fetal cortical tissue. “About 70% of cellular gene expression was conserved between organoids and fetal cells, which is reassuring, but shows that there is still room for improvement.”
Next Steps
Despite these limitations, neuroscientists see a path forward for brain organoids.
“Right now, researchers should be going for the lowest-hanging fruit in terms of disease models,” said Steven Hyman, M.D., a Harvard University Distinguished Service Professor of Stem Cell and Regenerative Biology and director of the Broad Institute’s Stanley Center for Psychiatric Research. “These are the rare, inherited cases of autism or schizophrenia that you can trace back to one or two mutations.”
Hyman said that with such rare syndromes, the dysfunction likely occurs in the earliest stages of development. This dysfunction may be able recreated by organoid models. “We then need to test interventions to see if we can correct the deficiency in a reliable and interpretable way.” If research with these rare syndromes proves successful, then organoids might be ready to explore more complex neurodevelopmental disorders.
All researchers queried by Psychiatric News agreed that organoids will be valuable for studying disorders such as autism or schizophrenia in which genetics and fetal exposures like inflammation or infection plays a strong role. There was less consensus on the application of organoids to diseases such as depression or anxiety.
“I don’t think these models will be immediately useful for studying complex disorders with a social component, such as affective disorders,” Pasca said.
Vaccarino was more optimistic. “Our field has come so far in just a decade, so I don’t want to rule anything out,” she said. “Maybe some individual disorders will be difficult to model, but we can tackle broad psychiatric questions like the nature of resilience: why some people at high risk develop a disorder while others do not.”
“This field has shown a lot of creativity already, so I’m excited to see what comes next,” said Arlotta. “Organoids will always be imperfect, but they will play a big role in psychiatric research moving forward.”
Arlotta and the others stressed that brain organoids will not replace any existing research models. “We still need genetics, we still need mouse models, we still need access to postmortem tissue,” Hyman said. “If we want to reach a deeper understanding of the human brain, we need every model we can get.” ■
“Functional Cortical Neurons and Astrocytes From Human Pluripotent Stem Cells in 3D Culture” is posted here.
“Generation of Functional Human 3D Cortico-Motor Assembloids” is posted here.
“Cell Stress in Cortical Organoids Impairs Molecular Subtype Specification” is posted here.
“Modeling human cortical development in vitro using induced pluripotent stem cells” is posted here.