Potential Schizophrenia Medications Point to New Disease Model
Abstract
New views of the biology of schizophrenia are converging with the development of novel drugs targeting new brain mechanisms that might reduce symptoms without blocking the dopamine D2 receptor.
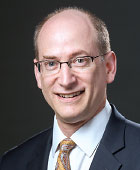
Despite progress in the treatment of schizophrenia, it remains one of the leading causes of disability worldwide.
The limited efficacy of antipsychotic treatments contributes to the burden of schizophrenia. A large meta-analysis found that only 23% of patients participating in clinical trials of schizophrenia had a good response, as defined by a 50% or greater reduction in symptoms on the Brief Psychiatric Rating Scale/Positive and Negative Symptom Score (BPRS/PANSS), and this was only approximately 10% better than the response rate on placebo.
Since the discovery of the antipsychotic effects of chlorpromazine, all medications approved by the Food and Drug Administration for the treatment of symptoms of schizophrenia have blocked the actions of dopamine at D2 receptors. This makes sense, as increased dopamine release in the antero-dorsal “associative” striatum associated with schizophrenia contributes to psychotic symptoms. Occupancy of these D2 receptors by D2 receptor antagonists normalizes their level of dopaminergic stimulation.
However, the extent to which schizophrenia symptoms are attributable to dopamine hyperactivity varies across patients, and many other mechanisms are implicated in the biology of schizophrenia that may not be addressed by blockade of D2 receptors.
This is an exciting moment in schizophrenia research—new views of the biology of schizophrenia are converging with novel drugs targeting new brain mechanisms that might treat symptoms without blocking the D2 receptor. This article will begin by reviewing these promising new medications and then suggesting a general neurodevelopmental framework that supports the exploration of these novel treatment mechanisms.
Targeting Glutamate
Several medications currently or previously under development target glutamate synaptic deficits that may be the earliest primary drivers of pathophysiology in schizophrenia. The mechanisms reviewed below include inhibition of the glycine transporter-1 (GlyT1), stimulation of metabotropic glutamate receptor-2 (mGluR2), stimulation of muscarinic M4 receptors, and stimulation of trace amine-associated receptor 1 (TAAR1). As will be reviewed below, stimulation of mGluR2, M4, and TAAR1 receptors share the ability to reduce striatal dopamine release and cortico-striatal glutamate release via mechanisms summarized in Figure 1.
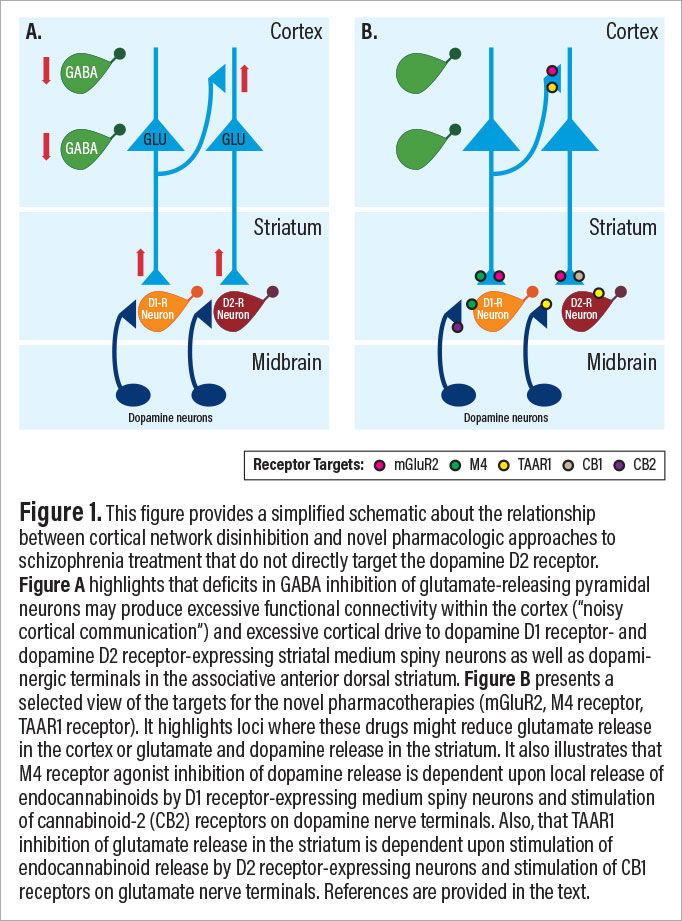
There has been extensive testing of strategies to enhance the stimulation of the glycine co-agonist site of the N-methyl-D-aspartate (NMDA) glutamate receptor. Studies have evaluated amino acid agonists (glycine, D-serine, D-alanine), a modified amino acid partial agonist (D-cycloserine), blocking the uptake of glycine (GlyT1), blocking the uptake of D-serine (ASCT1), and inhibiting the enzyme that metabolizes D-serine (D-amino acid oxidase, DAO). Overall, these substances have either not been adequately evaluated or they have not consistently produced sufficient clinical improvement to result in FDA-approved clinical indications.
One promising recent finding, though, suggested that GlyT1 inhibitors might reduce neuroplasticity deficits associated with schizophrenia. Although a Roche GlyT inhibitor, bitopertin, failed to show efficacy in phase 3 trials after generating promising results in phase 2, a recent study with the GlyT1 inhibitor BI425809 did find promising effects on cognitive deficits. This 12-week study (n=509, approximately 85 patients per group) reported dose-related performance improvements on the Matric Consensus Cognitive Battery, particularly improvements in processing speed as measured by the Trail-Making Task, but no significant changes in symptoms. They also suggested that improvements were greater in patients who were not taking benzodiazepines. However, GlyT1 inhibitor studies have yet to be conducted in prodromal or early course patients, groups for which an illness phase-related model would predict benefit. The only data to date come from a promising open-label study of glycine showing reductions in symptoms in patients with prodromal symptoms in ultra-high-risk patients.
Arguably the first novel medication tested to show efficacy in reducing psychosis in schizophrenia without blocking D2 receptors was the metabotropic glutamate receptor 2/3 (mGlur2/3) agonist LY2140023, a prodrug of LY404039. mGluR2 receptors serve both as inhibitory auto- and heteroreceptors for glutamate, where mGluR2 agonists inhibit glutamate release in the cortex and in the striatum, and where these drugs secondarily inhibit dopamine release (see Figure 1B). After an initial positive study as monotherapy for schizophrenia, subsequent studies did not show efficacy. However, a secondary analysis found that LY21340023 was helpful early in the course of illness, when cortical functional hyperconnectivity predominates, and was ineffective or even worsened symptoms in chronic illness, when functional connectivity deficits associated with synaptic losses might be exacerbated by mGluR2/3 agonism. Thus, while mGluR2/3 agonists may not have general efficacy for symptoms of schizophrenia, it may some day play a treatment role within a precision medicine strategy.
Promising results from two recent phase 2 clinical trials identified two innovative strategies for treating schizophrenia symptoms without blocking D2 receptors: stimulation of muscarinic M1/M4 receptors and agonism of TAAR1. There has been longstanding interest in the therapeutic role of M4 receptor agonism after clozapine, its metabolite desmethylclozapine, and xanomeline. All were shown to exhibit M4 agonist or partial agonist activity. While the therapeutic effects of clozapine are well known, a pilot study of xanomeline and more recently a larger study of xanomeline-tropsium (n=90, placebo n=92) combining xanomeline and a peripheral muscarinic receptor antagonist intended to improve xanomeline tolerability (trospium) showed evidence of efficacy for positive and negative symptoms. A TAAR1 and 5HT1A agonist, SEP-363856, also showed efficacy as monotherapy for both positive and negative symptoms associated with schizophrenia in a large phase 2 trial of SEP-363856 (n=125, placebo n=120).
These novel antipsychotic medications have been hypothesized to produce clinical improvement through both dopaminergic and glutamatergeric mechanisms (see Figure 1B). For example, both M4 agonists and TAAR1 agonists are reported to reduce dopamine release. As has been reviewed, M4 agonists appear to reduce dopamine release by stimulating M4 receptors located on dopamine-1 receptor-bearing medium spiny neurons (MSN) in the striatum that are postsynaptic to dopamine projections. M4 receptor stimulation in these MSNs causes the release of endocannabinoids, particularly 2-AG. 2-AG then back diffuses across the synapse to stimulate CB2 cannabinoid receptors and suppress dopamine release. M4 receptors are also located on several other cellular elements in the striatum including cholinergic interneurons and glutamatergic axon terminals of neurons projecting from the cortex and thalamus.
TAAR1 agonism inhibits striatal dopamine release through several mechanisms. TAAR1 can co-localize with dopamine D2 receptors, form D2-TAAR1 heterodimers, and facilitate D2 autoreceptor inhibition of dopamine release. TAAR1 facilitation of D2 receptor function also triggers endocannabinoid release from MSNs and stimulation of cannabinoid CB1 receptors on cortical projections to the striatum, inhibiting glutamate release. Also, TAAR1 is present in layer V pyramidal neurons in the prefrontal cortex (PFC), where it may affect the balance of AMPA and NMDA glutamate receptor signaling and buffer stress related effects on PFC function.
Targeting Medications to Illness Stages
The three new medications that reduce psychosis without blocking dopamine D2 receptors are all believed to reduce the release of dopamine and glutamate in cortico-striatal circuits (see Figure 1B). This constitutes a striking mechanistic convergence at the circuit level. We previously suggested that the neurobiology of schizophrenia evolves over the lifespan of the individual and that medications might be developed that targeted particular illness phases.
This model suggests that glutamate synaptic deficits are present prenatally and that they compromise the development and maintenance of GABA interneurons. During adolescence, the resulting deficits in GABA signaling disinhibit cortical networks, giving rise to cortical functional hyperconnectivity, noisy cortical information processing, less precise cortical representations of information, and hyperactivity of cortical projections to the striatum, contributing to the dorsal striatal dopaminergic hyperactivity that is associated with psychosis (see Figure 1A). We posit that in chronic schizophrenia, the network disinhibition triggers a homeostatic downregulation of glutamate synaptic structure and function that exacerbates the impact of programmed synaptic elimination and other mechanisms through which synaptic elimination might be accelerated in schizophrenia, such as enhanced expression of complement C4A.
Implicit in this model is that, across development, the pathology of schizophrenia not only progresses but it becomes more complex; this makes it more difficult to treat the full spectrum of schizophrenia symptoms with single agents. The key therapeutic implication of this model, which hypothesizes both progressive and increasingly complex pathophysiology, is that a given treatment is likely to have its greatest impact at the earliest phase of illness when relevant circuit mechanisms targeted by that treatment are expressed. For example, a given treatment, such as an mGluR2 agonist, might be effective early in the course of illness when it compensates for deficits in cortical inhibition, but it might lose efficacy as the illness progresses and the same drug now exacerbates the impact of synaptic deficits.
In summary, there are now at least four promising new medications under study for the treatment of schizophrenia. The fact that three of these new drugs inhibit the release of glutamate and dopamine draws our attention to pathophysiologic models for schizophrenia that encompass excessive glutamate and dopamine release. The model considered here suggests that excessive glutamate and dopamine release emerges in the high-risk state and persists through the early course of schizophrenia.
Later in the illness, synaptic loss complicates both the biology and treatment of this illness. This illness phase-specific approach to pharmacotherapy is a framework for increasing the precision of schizophrenia pharmacotherapy. However, the developmental model proposed is a general one, and we recognize that there are important differences in the biology of schizophrenia across patients that might account for why an individual patient might not fit this model. The approach outlined above would be more effective were it based on biomarkers of circuit function in individual patients. This type of biomarker-driven approach might yield even more precise matching of inhibitory treatments to evidence of network disinhibition or dopaminergic hyperactivity in individual patients. For example, genomic biomarkers might also drive the development of novel therapeutics, particularly those patients with specific rare loss of function mutations that produce substantial increases in schizophrenia. If individuals with those mutations could be identified as early as possible, it would create the possibility of instituting specific medication treatments or gene therapies well in advance of the onset of psychotic symptoms. As so frequently happens in psychiatry, discovering pharmacotherapies that work through novel mechanisms focuses our attention on new aspects of the biology and new opportunities for the treatment of this disorder.
This work was supported by UO1 MH121766-01. ■
References
F.J. Charlson, A.J. Ferrari, D.F. Santomauro, S. Diminic, E. Stockings, J.G. Scott, J.J. McGrath, H.A. Whiteford, Global Epidemiology and Burden of Schizophrenia: Findings From the Global Burden of Disease Study 2016, Schizophr Bull 44(6) (2018) 1195-1203.
S. Leucht, C. Leucht, M. Huhn, A. Chaimani, D. Mavridis, B. Helfer, M. Samara, M. Rabaioli, S. Bächer, A. Cipriani, J.R. Geddes, G. Salanti, J.M. Davis, Sixty Years of Placebo-Controlled Antipsychotic Drug Trials in Acute Schizophrenia: Systematic Review, Bayesian Meta-Analysis, and Meta-Regression of Efficacy Predictors, Am J Psychiatry 174(10) (2017) 927-942.
J.J. Weinstein, M.O. Chohan, M. Slifstein, L.S. Kegeles, H. Moore, A. Abi-Dargham, Pathway-Specific Dopamine Abnormalities in Schizophrenia, Biol Psychiatry 81(1) (2017) 31-42.
M. Laruelle, A. Abi-Dargham, Dopamine as the wind of the psychotic fire: new evidence from brain imaging studies, Journal of Psychopharmacology. 13(4) (1999) 358-71.
J.H. Krystal, A. Anticevic, Toward illness phase-specific pharmacotherapy for schizophrenia, Biol Psychiatry 78(11) (2015) 738-40.
R.R. Girgis, A.W. Zoghbi, D.C. Javitt, J.A. Lieberman, The past and future of novel, non-dopamine-2 receptor therapeutics for schizophrenia: A critical and comprehensive review, J Psychiatr Res 108 (2019) 57-83.
D.C. D’Souza, R.E. Carson, N. Driesen, J. Johannesen, M. Ranganathan, J.H. Krystal, Dose-Related Target Occupancy and Effects on Circuitry, Behavior, and Neuroplasticity of the Glycine Transporter-1 Inhibitor PF-03463275 in Healthy and Schizophrenia Subjects, Biol Psychiatry 84(6) (2018) 413-421.
W.W. Fleischhacker, J. Podhorna, M. Gröschl, S. Hake, Y. Zhao, S. Huang, R.S.E. Keefe, M. Desch, R. Brenner, D.P. Walling, E. Mantero-Atienza, K. Nakagome, S. Pollentier, Efficacy and safety of the novel glycine transporter inhibitor BI 425809 once daily in patients with schizophrenia: a double-blind, randomised, placebo-controlled phase 2 study, The lancet. Psychiatry 8(3) (2021) 191-201.
S.W. Woods, B.C. Walsh, K.A. Hawkins, T.J. Miller, J.R. Saksa, D.C. D’Souza, G.D. Pearlson, D.C. Javitt, T.H. McGlashan, J.H. Krystal, Glycine treatment of the risk syndrome for psychosis: report of two pilot studies, European neuropsychopharmacology: the journal of the European College of Neuropsychopharmacology 23(8) (2013) 931-40.
B.J. Kinon, B.A. Millen, L. Zhang, D.L. McKinzie, Exploratory Analysis for a Targeted Patient Population Responsive to the Metabotropic Glutamate 2/3 Receptor Agonist Pomaglumetad Methionil in Schizophrenia, Biol Psychiatry (2015).
K.A. Johnson, Y. Mateo, D.M. Lovinger, Metabotropic glutamate receptor 2 inhibits thalamically-driven glutamate and dopamine release in the dorsal striatum, Neuropharmacology 117 (2017) 114-123.
A.L. Pehrson, B. Moghaddam, Impact of metabotropic glutamate 2/3 receptor stimulation on activated dopamine release and locomotion, Psychopharmacology (Berl) 211(4) (2010) 443-55.
S.T. Patil, L. Zhang, F. Martenyi, S.L. Lowe, K.A. Jackson, B.V. Andreev, A.S. Avedisova, L.M. Bardenstein, I.Y. Gurovich, M.A. Morozova, S.N. Mosolov, N.G. Neznanov, A.M. Reznik, A.B. Smulevich, V.A. Tochilov, B.G. Johnson, J.A. Monn, D.D. Schoepp, Activation of mGlu2/3 receptors as a new approach to treat schizophrenia: a randomized Phase 2 clinical trial, Nat Med 13(9) (2007) 1102-7.
X.P. Zeng, F. Le, E. Richelson, Muscarinic m4 receptor activation by some atypical antipsychotic drugs, Eur J Pharmacol 321(3) (1997) 349-54.
S. Gigout, S. Wierschke, C. Dehnicke, R.A. Deisz, Different pharmacology of N-desmethylclozapine at human and rat M2 and M 4 mAChRs in neocortex, Naunyn Schmiedebergs Arch Pharmacol 388(5) (2015) 487-96.
S.K. Brannan, S. Sawchak, A.C. Miller, J.A. Lieberman, S.M. Paul, A. Breier, Muscarinic Cholinergic Receptor Agonist and Peripheral Antagonist for Schizophrenia, N Engl J Med 384(8) (2021) 717-726.
K.S. Koblan, J. Kent, S.C. Hopkins, J.H. Krystal, H. Cheng, R. Goldman, A. Loebel, A Non-D2-Receptor-Binding Drug for the Treatment of Schizophrenia, N Engl J Med 382(16) (2020) 1497-1506.
M.S. Moehle, P.J. Conn, Roles of the M(4) acetylcholine receptor in the basal ganglia and the treatment of movement disorders, Mov Disord 34(8) (2019) 1089-1099.
Y. Pei, A. Asif-Malik, J.J. Canales, Trace Amines and the Trace Amine-Associated Receptor 1: Pharmacology, Neurochemistry, and Clinical Implications, Frontiers in Neuroscience 10 (2016) 148.
A. Alvarsson, X. Zhang, T.L. Stan, N. Schintu, B. Kadkhodaei, M.J. Millan, T. Perlmann, P. Svenningsson, Modulation by Trace Amine-Associated Receptor 1 of Experimental Parkinsonism, L-DOPA Responsivity, and Glutamatergic Neurotransmission, J Neurosci 35(41) (2015) 14057-69.
S. Espinoza, G. Lignani, L. Caffino, S. Maggi, I. Sukhanov, D. Leo, L. Mus, M. Emanuele, G. Ronzitti, A. Harmeier, L. Medrihan, T.D. Sotnikova, E. Chieregatti, M.C. Hoener, F. Benfenati, V. Tucci, F. Fumagalli, R.R. Gainetdinov, TAAR1 Modulates Cortical Glutamate NMDA Receptor Function, Neuropsychopharmacology 40(9) (2015) 2217-27.
Y. Zhang, J.T. Li, H. Wang, W.P. Niu, C.C. Zhang, Y. Zhang, X.D. Wang, T.M. Si, Y.A. Su, Role of trace amine associated receptor 1 in the medial prefrontal cortex in chronic social stress-induced cognitive deficits in mice, Pharmacol Res 167 (2021) 105571.
J.H. Krystal, A. Anticevic, G.J. Yang, G. Dragoi, N.R. Driesen, X.J. Wang, J.D. Murray, Impaired Tuning of Neural Ensembles and the Pathophysiology of Schizophrenia: A Translational and Computational Neuroscience Perspective, Biol Psychiatry 81(10) (2017) 874-885.
A. Sekar, A.R. Bialas, H. de Rivera, A. Davis, T.R. Hammond, N. Kamitaki, K. Tooley, J. Presumey, M. Baum, V. Van Doren, G. Genovese, S.A. Rose, R.E. Handsaker, M.J. Daly, M.C. Carroll, B. Stevens, S.A. McCarroll, Schizophrenia risk from complex variation of complement component 4, Nature 530(7589) (2016) 177-83.
T. Singh, J.T.R. Walters, M. Johnstone, D. Curtis, J. Suvisaari, M. Torniainen, E. Rees, C. Iyegbe, D. Blackwood, A.M. McIntosh, G. Kirov, D. Geschwind, R.M. Murray, M. Di Forti, E. Bramon, M. Gandal, C.M. Hultman, P. Sklar, A. Palotie, P.F. Sullivan, M.C. O’Donovan, M.J. Owen, J.C. Barrett, The contribution of rare variants to risk of schizophrenia in individuals with and without intellectual disability, Nat Genet 49(8) (2017) 1167-1173.
S.E. Legge, M.L. Santoro, S. Periyasamy, A. Okewole, A. Arsalan, K. Kowalec, Genetic architecture of schizophrenia: a review of major advancements, Psychol Med (2021) 1-10.
Disclosure Statement of John H. Krystal, M.D.
The Individual Consultant Agreements listed below are less than $10,000 per year.
Aptinyx, Atai Life Sciences, AstraZeneca Pharmaceuticals, Biogen, Idec, MA, Biomedisyn Corporation, Bionomics, Limited (Australia), Boehringer Ingelheim International, Cadent Therapeutics, Clexio Bioscience, Ltd., COMPASS Pathways, Limited, United Kingdom, Concert Pharmaceuticals, Epiodyne, EpiVario, Greenwich Biosciences, Heptares Therapeutics, Limited (UK), Janssen Research & Development, Jazz Pharmaceuticals, Otsuka America Pharmaceutical, Perception Neuroscience Holdings, Spring Care, Sunovion Pharmaceuticals, Takeda Industries, Taisho Pharmaceutical Co., Ltd.
Scientific Advisory Board
Biohaven Pharmaceuticals, BioXcel Therapeutics, Inc. (Clinical Advisory Board), Cadent Therapeutics, Inc. (Clinical Advisory Board), Cerevel Therapeutics, LLC., EpiVario, Inc, Eisai, Jazz Pharmaceuticals, Lohocla Research Corporation, Novartis Pharmaceuticals Corporation, PsychoGenics, RBNC Therapeutics, Tempero Bio, Terran Biosciences.
Board of Directors
Freedom Biosciences, Inc.
Stock
Biohaven Pharmaceuticals, Sage Pharmaceuticals, Spring Care Inc.
Stock Options
Biohaven Pharmaceuticals Medical Sciences, EpiVario, RBNC Therapeutics Inc., Terran Biosciences, Tempero Bio, Inc.
Income Greater than $10,000
Editorial Board
Editor, Biological Psychiatry